5 Conditions Of Hardy Weinberg Equilibrium
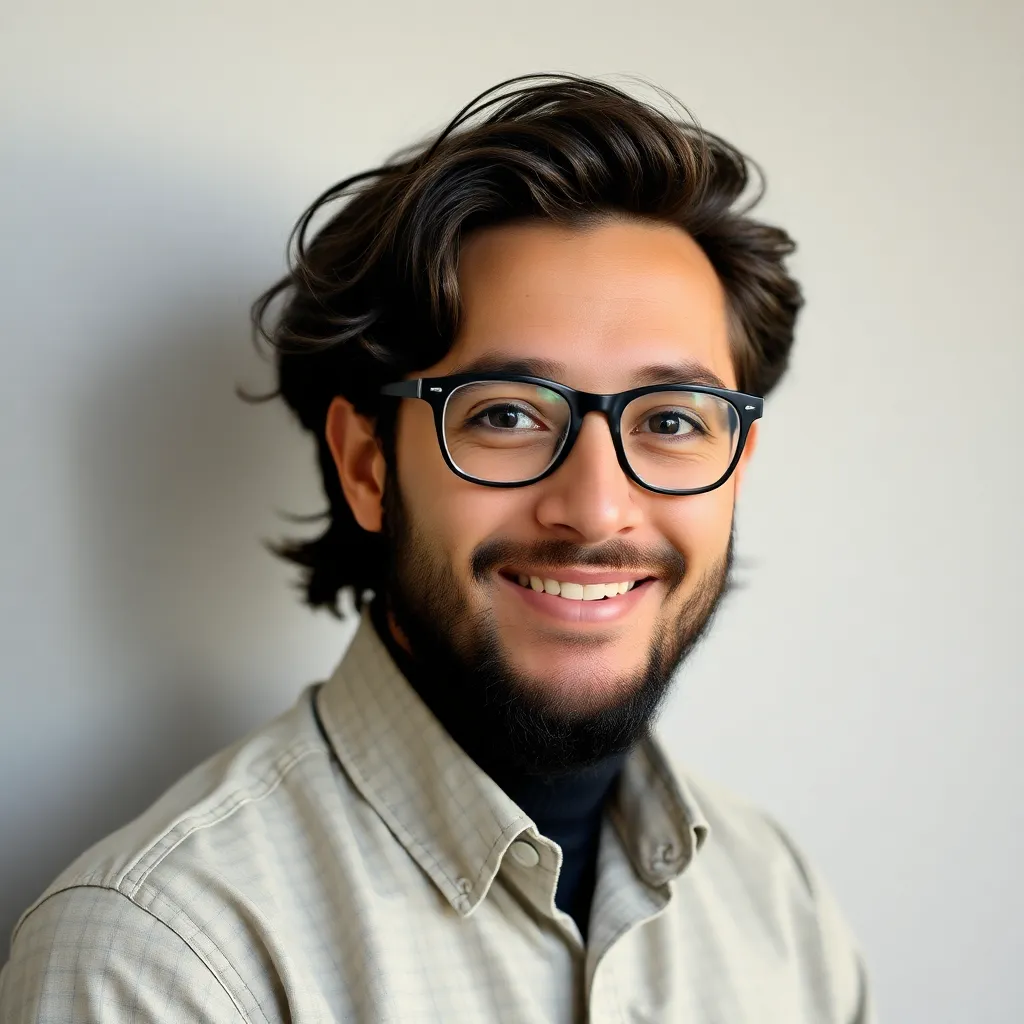
Juapaving
Apr 11, 2025 · 7 min read

Table of Contents
5 Conditions of Hardy-Weinberg Equilibrium: A Deep Dive
The Hardy-Weinberg principle, also known as the Hardy-Weinberg equilibrium, is a fundamental concept in population genetics. It describes the theoretical conditions under which allele and genotype frequencies in a population will remain constant from generation to generation, thus experiencing no evolution. Understanding these conditions is crucial for comprehending evolutionary processes and how populations change over time. This principle serves as a null hypothesis; deviations from it indicate that evolutionary forces are at play. Let's delve into the five key conditions that must be met for Hardy-Weinberg equilibrium to hold true.
The Five Pillars of Hardy-Weinberg Equilibrium
The Hardy-Weinberg equilibrium rests upon five fundamental assumptions. If any of these conditions are violated, the allele and genotype frequencies will change, and the population will evolve. These conditions are:
- No Mutation: The rate of mutation must be negligible. Mutations introduce new alleles into the population, altering allele frequencies.
- Random Mating: Individuals must mate randomly, without any preference for certain genotypes. Non-random mating, such as assortative mating (mating with similar individuals) or disassortative mating (mating with dissimilar individuals), can alter genotype frequencies.
- No Gene Flow: There should be no migration of individuals into or out of the population. Gene flow introduces or removes alleles, changing allele frequencies.
- No Genetic Drift: The population must be large enough to avoid random fluctuations in allele frequencies due to chance events. Genetic drift is particularly impactful in small populations.
- No Natural Selection: All genotypes must have equal survival and reproductive rates. Natural selection favors certain genotypes, leading to changes in allele frequencies.
Let's explore each condition in detail:
1. No Mutation: The Stability of the Gene Pool
Mutations are changes in the DNA sequence that can result in new alleles. These alterations can range from single nucleotide polymorphisms (SNPs) to larger chromosomal rearrangements. A constant influx of new mutations disrupts the equilibrium by introducing novel genetic variation. The rate of mutation is typically low, but over many generations, the cumulative effect of mutations can significantly alter allele frequencies. For Hardy-Weinberg equilibrium to hold, the mutation rate must be so low as to be essentially negligible. In real-world populations, mutation is constantly occurring, but its impact on equilibrium is often minor compared to other evolutionary forces.
Understanding the Impact of Mutation Rates
The rate of mutation varies across species and even within the genome of a single organism. Some genes are more prone to mutations than others due to their sequence context or location within the genome. Understanding mutation rates is crucial in evolutionary biology, allowing scientists to model the impact of mutations on population genetic structure and estimate evolutionary timescales. While small mutation rates don't drastically affect allele frequencies in a short period, their cumulative effect over extensive evolutionary time is undeniably significant.
2. Random Mating: The Absence of Mate Choice
Random mating, also known as panmixia, implies that individuals mate without any preference for particular genotypes. Each individual has an equal chance of mating with any other individual in the population, irrespective of their genotype. This ensures that the frequency of different genotypes in the offspring generation accurately reflects the allele frequencies in the parent generation.
The Distorting Effects of Non-Random Mating
However, random mating is rarely observed in natural populations. Many species exhibit various forms of non-random mating:
- Assortative mating: Individuals with similar phenotypes tend to mate more frequently. This leads to an increase in homozygosity (having two identical alleles for a gene) and can result in a higher frequency of homozygous genotypes than predicted by Hardy-Weinberg equilibrium.
- Disassortative mating: Individuals with dissimilar phenotypes tend to mate more frequently. This increases heterozygosity (having two different alleles for a gene) and leads to a higher frequency of heterozygous genotypes.
- Inbreeding: Mating between closely related individuals increases the likelihood of offspring inheriting identical alleles from both parents, leading to a higher frequency of homozygous genotypes and a reduction in genetic diversity.
These deviations from random mating significantly impact genotype frequencies, thus violating the Hardy-Weinberg equilibrium.
3. No Gene Flow: The Closed Population
Gene flow refers to the movement of alleles between populations. This can occur through migration of individuals or the dispersal of gametes (e.g., pollen in plants). Gene flow introduces new alleles into a population, altering allele frequencies, and potentially increasing genetic variation within the population. Conversely, it can also lead to homogenization of allele frequencies between populations. The absence of gene flow is essential for Hardy-Weinberg equilibrium as it ensures that the allele frequencies within a population are not affected by external influences.
Gene Flow's Influence on Population Dynamics
Gene flow can have significant impacts on the evolutionary trajectory of a population. For example, if a population is adapted to a specific environment and receives a significant influx of alleles from a different population adapted to a different environment, this can disrupt the local adaptation and potentially reduce the fitness of the population. Understanding gene flow dynamics is crucial in conservation biology, helping to predict the effects of habitat fragmentation and manage gene flow to maintain genetic diversity in threatened species.
4. No Genetic Drift: The Power of Large Population Size
Genetic drift refers to random fluctuations in allele frequencies that occur due to chance events, particularly in small populations. Imagine a population with only a few individuals carrying a specific allele. Due to chance events (such as random mating or survival), this allele might be lost from the population entirely, even if it's not selectively disadvantageous. The smaller the population, the greater the impact of genetic drift. In contrast, large populations are buffered against these random fluctuations; allele frequencies are relatively stable. Hardy-Weinberg equilibrium requires a sufficiently large population size to minimize the effects of genetic drift.
The Bottleneck Effect and Founder Effect
Two significant examples of genetic drift are the bottleneck effect and the founder effect.
- Bottleneck effect: A population drastically reduces in size due to a catastrophic event (e.g., natural disaster, disease outbreak). This significantly reduces genetic diversity and can lead to random changes in allele frequencies.
- Founder effect: A small group of individuals establishes a new population, carrying only a subset of the original population's genetic variation. This new population may have different allele frequencies than the original population, and genetic diversity is typically reduced.
5. No Natural Selection: Equal Fitness for All Genotypes
Natural selection is the process by which individuals with certain heritable traits are more likely to survive and reproduce than individuals without those traits. These traits confer a selective advantage, increasing the frequency of the beneficial alleles in subsequent generations. For Hardy-Weinberg equilibrium, all genotypes must have equal fitness, meaning that they all have the same survival and reproductive rates. If certain genotypes have higher fitness, their frequencies will increase, and the population will evolve, violating the equilibrium.
Understanding Fitness Landscapes
Fitness is not an absolute value; it's relative to the other genotypes in the population and the prevailing environmental conditions. A genotype might be highly fit in one environment but less fit in another. The concept of a "fitness landscape" helps visualize the relationship between genotype and fitness, providing a framework for understanding how natural selection shapes populations.
Conclusion: The Hardy-Weinberg Equilibrium as a Benchmark
The Hardy-Weinberg principle is a powerful tool for understanding evolutionary processes. It serves as a null hypothesis: if a population deviates from Hardy-Weinberg equilibrium, it indicates that evolutionary forces are at play. By identifying which conditions are violated, we can gain insights into the mechanisms driving evolutionary change. While the ideal conditions for Hardy-Weinberg equilibrium rarely exist in nature, understanding this principle provides a foundation for analyzing the complex dynamics of real-world populations and for predicting how populations might evolve under different circumstances. It’s a crucial cornerstone in the study of population genetics and evolutionary biology, offering a framework for interpreting observed genetic variation and predicting future evolutionary trends. The ongoing exploration and refinement of this principle continue to enhance our comprehension of the intricate mechanisms that shape biodiversity on our planet.
Latest Posts
Latest Posts
-
What Unit Is Velocity Measured In
Apr 18, 2025
-
Derivative Of Square Root X 2 1
Apr 18, 2025
-
How To Find The Kinetic Friction
Apr 18, 2025
-
Which Expression Is Equivalent To St 6
Apr 18, 2025
-
How Many Yards Is 500 Feet
Apr 18, 2025
Related Post
Thank you for visiting our website which covers about 5 Conditions Of Hardy Weinberg Equilibrium . We hope the information provided has been useful to you. Feel free to contact us if you have any questions or need further assistance. See you next time and don't miss to bookmark.