3 Functions Of The Plasma Membrane
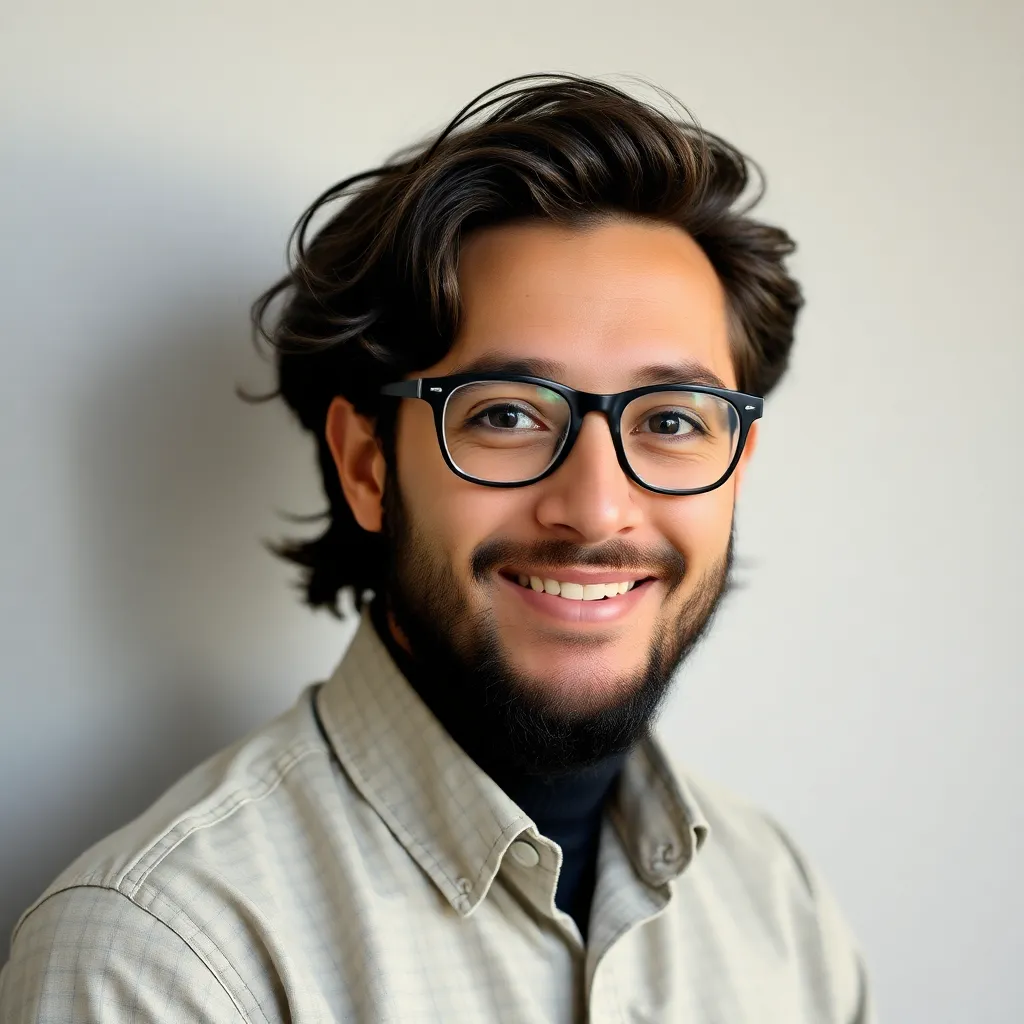
Juapaving
May 09, 2025 · 8 min read
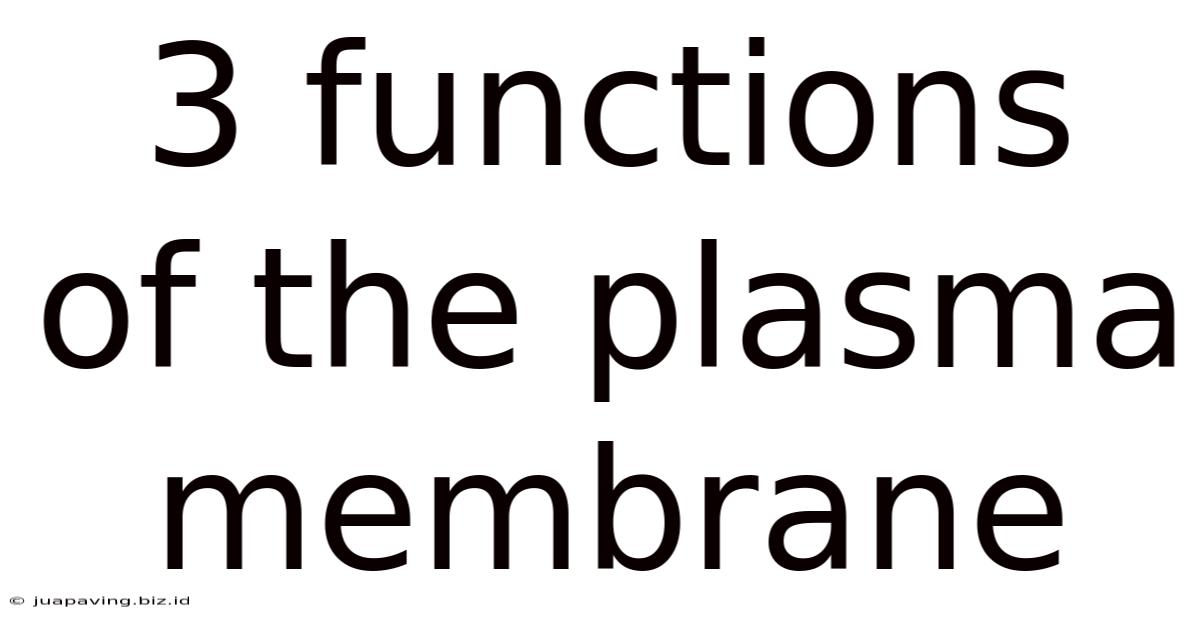
Table of Contents
3 Essential Functions of the Plasma Membrane: A Deep Dive
The plasma membrane, also known as the cell membrane, is a vital component of all living cells. This selectively permeable barrier isn't just a passive enclosure; it's a dynamic structure orchestrating a multitude of crucial cellular processes. While its functions are interconnected and complex, we can highlight three primary roles: transport, cell signaling, and cell adhesion. Understanding these functions is key to grasping the intricate workings of life itself.
1. Transport: The Gatekeeper of the Cell
The plasma membrane acts as a highly selective gatekeeper, meticulously regulating the passage of substances into and out of the cell. This control is paramount for maintaining cellular homeostasis – the stable internal environment necessary for survival. The transport mechanisms can be broadly categorized into passive and active transport.
1.1 Passive Transport: Moving with the Flow
Passive transport processes don't require energy expenditure by the cell. Instead, they rely on the inherent properties of the molecules being transported and the concentration gradients across the membrane. Several types of passive transport exist:
-
Simple Diffusion: This is the simplest form, where small, nonpolar molecules like oxygen (O2) and carbon dioxide (CO2) move directly across the lipid bilayer from an area of high concentration to an area of low concentration. This movement continues until equilibrium is reached. The rate of diffusion is influenced by factors such as the concentration gradient, temperature, and the size and polarity of the molecule.
-
Facilitated Diffusion: Larger or polar molecules, which cannot readily cross the lipid bilayer, require assistance. This is where membrane proteins step in. These proteins act as channels or carriers, providing specific pathways for these molecules to move down their concentration gradients. Channel proteins form hydrophilic pores allowing the passage of ions or small polar molecules, while carrier proteins bind to the molecule and undergo a conformational change to transport it across the membrane. Glucose transport is a classic example of facilitated diffusion. The rate of facilitated diffusion is often influenced by the number of transport proteins available. Saturation can occur when all transport proteins are occupied.
-
Osmosis: This specific type of passive transport refers to the movement of water across a selectively permeable membrane from a region of high water concentration (low solute concentration) to a region of low water concentration (high solute concentration). Osmosis is crucial for maintaining cell turgor pressure in plants and regulating fluid balance in cells. The osmotic pressure, the pressure required to prevent osmosis, is a crucial factor in cellular function and survival. Understanding osmotic pressure is vital for comprehending phenomena like plasmolysis (cell shrinkage due to water loss) and cytolysis (cell bursting due to water influx).
1.2 Active Transport: Energy-Dependent Movement
Unlike passive transport, active transport requires energy, typically in the form of ATP (adenosine triphosphate), to move molecules against their concentration gradients—from an area of low concentration to an area of high concentration. This uphill movement is essential for maintaining concentration gradients vital for various cellular processes.
-
Primary Active Transport: This involves the direct use of ATP to move molecules. A prime example is the sodium-potassium pump (Na+/K+ ATPase), which maintains the electrochemical gradient across the cell membrane by pumping sodium ions (Na+) out of the cell and potassium ions (K+) into the cell. This gradient is crucial for nerve impulse transmission and muscle contraction. The pump's activity is tightly regulated to maintain cellular homeostasis.
-
Secondary Active Transport: This utilizes the energy stored in electrochemical gradients created by primary active transport. Instead of directly using ATP, it couples the movement of one molecule down its concentration gradient to the movement of another molecule against its gradient. For example, the absorption of glucose in the intestines utilizes the sodium gradient established by the sodium-potassium pump. This process is also known as co-transport or symport if both molecules move in the same direction, and counter-transport or antiport if they move in opposite directions.
1.3 Vesicular Transport: Bulk Movement
Vesicular transport involves the movement of large molecules or groups of molecules across the membrane using membrane-bound vesicles. This process is crucial for transporting macromolecules such as proteins and polysaccharides.
-
Exocytosis: This process involves the fusion of intracellular vesicles with the plasma membrane, releasing their contents outside the cell. This is crucial for secretion of hormones, neurotransmitters, and waste products. The precise targeting and fusion of vesicles are tightly regulated processes.
-
Endocytosis: This is the reverse of exocytosis, where the plasma membrane invaginates to engulf extracellular material, forming a vesicle that is then internalized. Several types of endocytosis exist, including phagocytosis (cell eating), pinocytosis (cell drinking), and receptor-mediated endocytosis (specific uptake of molecules bound to receptors on the cell surface). Receptor-mediated endocytosis allows for highly selective uptake of specific molecules, ensuring efficient internalization of necessary substances.
2. Cell Signaling: Communication is Key
The plasma membrane is not just a barrier; it's also a crucial platform for cell communication. It's densely populated with receptors that bind to specific signaling molecules, initiating intracellular cascades that regulate a wide array of cellular responses. These signaling pathways are incredibly complex and tightly regulated, ensuring appropriate responses to external stimuli.
2.1 Receptor Proteins: Receiving the Message
Receptor proteins embedded within the plasma membrane are responsible for recognizing and binding to specific signaling molecules (ligands). These ligands can include hormones, neurotransmitters, growth factors, and other extracellular messengers. The binding of a ligand to its receptor triggers a conformational change in the receptor, initiating a signaling cascade within the cell.
2.2 Signal Transduction Pathways: Relaying the Message
Once a ligand binds to its receptor, a series of intracellular events, collectively known as signal transduction, ensues. These pathways often involve a complex network of protein kinases, second messengers (e.g., cAMP, IP3), and other signaling molecules. The signal is amplified and relayed to specific target molecules within the cell, ultimately leading to a cellular response, which can range from changes in gene expression to alterations in cell metabolism and motility. The specificity of these pathways is crucial for ensuring that the correct response is elicited in response to the specific signal.
2.3 Cellular Responses: Acting on the Message
The ultimate outcome of cell signaling is a change in cellular behavior. These responses can be diverse and include changes in gene expression, enzyme activity, cell growth, differentiation, apoptosis (programmed cell death), and cell movement. The diversity and complexity of signaling pathways allow cells to respond to a wide range of stimuli in a highly specific and regulated manner. Disruptions in these signaling pathways can contribute to various diseases.
3. Cell Adhesion: Sticking Together
The plasma membrane plays a vital role in cell adhesion – the process by which cells adhere to each other and to the extracellular matrix (ECM). This adhesion is crucial for maintaining tissue integrity, coordinating cell behavior, and enabling cell migration during development and wound healing.
3.1 Cell-Cell Adhesion: Connecting the Dots
Cells utilize various adhesion molecules to adhere to each other. These molecules, often transmembrane proteins, interact with similar molecules on adjacent cells. Some key types of cell-cell adhesion molecules include:
-
Cadherins: Calcium-dependent adhesion molecules that mediate strong cell-cell adhesion, crucial for the formation and maintenance of tissues and organs. Different types of cadherins are expressed in different tissues, contributing to tissue-specific adhesion.
-
Integrins: Transmembrane receptors that bind to both ECM components and intracellular cytoskeletal proteins. They play a crucial role in cell adhesion, migration, and signaling.
-
Immunoglobulins: A large family of adhesion molecules involved in various aspects of cell adhesion and immune responses.
3.2 Cell-Matrix Adhesion: Anchoring to the Surroundings
Cells also adhere to the ECM, a complex network of proteins and polysaccharides surrounding cells. This adhesion is mediated by integrins, which bind to ECM components like fibronectin and laminin. This interaction provides structural support, influences cell shape and behavior, and transmits signals from the ECM to the cell interior. The strength and nature of cell-matrix adhesion are crucial for various physiological processes.
3.3 The Extracellular Matrix: Providing Support and Signaling
The ECM is a dynamic and complex structure that plays a crucial role in cell adhesion and signaling. It not only provides structural support but also influences cell behavior by presenting signals that regulate cell growth, differentiation, and migration. The composition and organization of the ECM vary depending on the tissue type, contributing to tissue-specific cell behavior.
Conclusion:
The plasma membrane, far from being a simple barrier, is a highly dynamic and sophisticated structure that orchestrates a multitude of essential cellular functions. Its roles in transport, cell signaling, and cell adhesion are intricately interconnected and crucial for maintaining cellular homeostasis, enabling communication, and establishing tissue integrity. Further research continues to unravel the complexity of these functions and their implications for health and disease. A deeper understanding of the plasma membrane's multifaceted roles is paramount to advancements in various fields, from medicine and biotechnology to fundamental biological research.
Latest Posts
Latest Posts
-
To What Domain Do Humans Belong
May 09, 2025
-
Which Of The Following Is Responsible For Muscle Relaxation
May 09, 2025
-
What Is The Oxidation Number Of Cl In Hclo4
May 09, 2025
-
29 6 As A Mixed Number
May 09, 2025
-
2 3 On A Number Line 0 1
May 09, 2025
Related Post
Thank you for visiting our website which covers about 3 Functions Of The Plasma Membrane . We hope the information provided has been useful to you. Feel free to contact us if you have any questions or need further assistance. See you next time and don't miss to bookmark.